Sizing a Battery-Based PV System
In addition to grid-direct PV systems, which I show you how to size in privious article, the other major PV system type you’ll likely have the opportunity to design and install is a battery-based system. You can install one of these systems on the grid (utility-interactive) or off the grid (stand-alone).
The basics of the design and installation process for both are similar, including the fact that sizing any battery-based system is equal parts art and science. The science comes into play when you analyze specific site information and calculate the power values of the battery bank and the array.
The art part is apparent when you work with clients to define realistic load profiles so you can size the entire system (people generally don’t like to admit to how much television they watch). The art of sizing battery-based systems is also apparent in the assumptions and estimations you have to make given that the variables affecting system sizing are always changing.
In this article, I show you how to approach sizing battery-based systems for utility-intertive and stand-alone applications. I walk you through the calculations to make when sizing the elements of either system and give you guidance on some of the assumptions and estimations you’ll need to make.
As with grid-direct systems, the customer’s budget and available space for a PV array are the initial considerations you should make. After all, you don’t want to spend a ton of time designing a system the client can’t afford or put on her property due to space constraints. Establish these limitations early on following the process I describe in privious article.
Looking at Loads in a Battery-Based System
A load is any piece of electrical equipment people want to use in their homes and offices. When sizing a battery-based system, you need to establish exactly what loads your client wants to run and how long she plans to run those loads. This information serves as the basis for all of your other calculations throughout the design process.
For utility-interactive, battery-based systems, the battery bank provides power for backup loads (loads that the client wants to have on regardless of the utility availability).
In this scenario, you have two load centers: the main distribution panel (MDP) and the backup subpanel. Any of the loads connected to the backup sub-panel will always be available, whereas the loads connected to the MDP will only be powered when the grid is present. For stand-alone, battery-based systems, the battery bank is designed to power all the electrical loads the client wants to run.
The sections that follow help you and your client take a critical look at the loads the battery bank will serve in both utility-interactive and stand-alone systems. They also help you figure out the energy needs of the loads that will rely (at least in part) on the battery bank.
Evaluating the loads that the battery bank must serve
After determining your client’s budget and available space, your next task when sizing any battery-based system is to evaluate the loads the batteries will be serving.
When I say loads, I mean all the loads — everything from the barely there energy drain (think small cellphone chargers) all the way up to the electrical hogs (think air-conditioning units).
When using batteries to power loads, you have to generate and store every watt-hour (Wh) used, which means you need to find out whether a more efficient alternative exists.
For example, compact fluorescent light bulbs (CFLs) produce the same amount of light as incandescent lights, but they use a lot less power. If you can convince your client to replace her incandescent bulbs with CFLs, her battery bank will be able to deliver power for more loads due to the reduced power requirements.
Ultimately, the less energy your client consumes, the less expensive the system will be to install and maintain. Following are some points to consider about the common loads powered entirely or partially by battery banks:
For well pumps: In many situations, a well pump is the sole source of water for a home. Well pumps can be large electrical loads with the potential to cause problems for the inverters and batteries in a battery-based system.
With advancements in inverter technologies, however, inverters are much better about running these large pumps. Look at the well pump’s power draw and try to determine the energy consumption. If the pump is being replaced or hasn’t been installed, try to work with the pump supplier to get the most energy-efficient version available.
For refrigeration and lighting: Refrigeration and lighting will be present in most homes or offices, so you need to be able to account for them accurately. In the next section, I show you how to account for the energy consumed by these appliances and how to make sure you design the battery system to handle these loads.
For phantom loads: The small loads that are on 24/7 are the so-called phantom loads. Many televisions and entertainment centers draw power even when they’re “off,” and chargers for small electronic devices and digital clocks incorporated in microwaves and stoves can cause major problems. If these small loads are always present, then the inverter can never turn off and must always supply power. Therefore, the inverter requires a small amount of power to produce a low level of power, causing it to operate at its worst efficiency level.
The solution to phantom loads? When no major loads are running, allow the inverter to go to sleep by unplugging the phantom loads. By removing these small loads, the inverter can go to sleep and wake up (and operate more efficiently) when the larger loads are turned on.
One type of load that should never be placed on a battery bank is anything that uses electricity to generate heat (these are called resistive loads). That rules out water heating, space heating, and electric stoves (incandescent lights also fall into this category).
In a stand-alone, battery-based system, petroleum-based fuels generally support resistive loads, with propane being one of the most common in off-grid applications.
For people connected to the grid with a utility-interactive, battery-based system, these loads can still be present; you just can’t back them up with the batteries. In a utility-interactive, battery-based system, loads served by the batteries should generally be kept to a minimum; the batteries should only supply power to loads that are truly necessary (which means you need to have a frank conversation with your client to help her evaluate what’s really necessary in her daily life).
This is because the utility grid is the primary power source, and the batteries are merely the backup power source. When talking about utility-interactive, battery-based systems, people often refer to loads the battery bank needs to back up when the grid fails as critical loads. Strike this phrase from your vocabulary when talking about these systems.
The National Electrical Code (NEC) defines critical loads as loads used for life support, public safety, and other truly vital functions. If you list the system as backing up critical loads when it really isn’t, a persnickety system inspector or plan checker may make your life harder.
When deciding what loads to power in utility-interactive, battery-based systems, the loads you should always consider include water-supply loads (well pumps and pressure pumps); lighting; refrigeration; and maybe one or two outlets to plug small appliances into, like a radio and a cellphone charger. (You must be careful, though; if you make it too easy to pull power from the battery bank, the system owner will do exactly that and then be disappointed in the lack of time that the batteries back up those “few” loads.)
For a stand-alone battery-based system, a load is just a plain old load, not a back- up load or a critical load. People who live in off-grid homes typically have major lifestyle differences from people who live in homes that are on the grid. They have to evaluate the necessity of anything that requires electricity to run because they don’t have unlimited access to electricity.
This doesn’t mean that your client can’t lead a “normal” life (whatever that means anymore); she just has to become selective in her electrical consumption. Some clients with stand-alone systems may want to run some loads straight from the DC electricity stored in the battery bank. Generally, the loads you can run from DC are limited to lighting and refrigeration.
These DC loads are nice in the sense that they can pull power directly from the battery bank without the help of an inverter, which increases the overall efficiency of the system because the loads use the same type of electricity produced by the PV array and stored in the batteries.
However, DC loads are found in specialty locations and must be matched to the voltage available from the batteries. Keep DC loads separate on your list of loads. When you have to account for system efficiencies, you’ll use the DC loads in your calculations.
Calculating the energy required during an outage for utility-interactive systems
For a utility-interactive, battery-based system, you need to know how much energy your client will need to use over a very short period of time. After all, most people experience power outages that are measured in hours (or a few days at the most).
On top of the short duration, typically only a few loads need to be backed up during an outage. If, however, your client insists on powering the entire house/office or a number of major loads, you need to incorporate a generator into the system design. I cover generators and how to include them later in this article.
When designing a utility-interactive, battery-based system, look at the loads the client wants to run during an outage and estimate how much energy they’ll consume during the specific amount of time required. You can do this by multiplying the total run watts for a load by the number of hours it needs to run during a utility outage to find the total energy consumption during the outage. Generally, 24 hours is enough, except for those clients who want to have multiple days of backup.
Determining the average daily energy consumption for stand-alone systems
After you’ve identified all the loads, you need to evaluate how much energy each load consumes in order to begin the process of sizing all the required components. Going through the load analysis may seem like a real pain, but if you don’t take the time to estimate each load’s energy consumption, the installed system will be either grossly under-or oversized for your client’s needs. Both situations result in a waste of time and money.
To determine the amount of energy consumed by each AC load in kilowatt- hours (kWh), you need to know the number of watts the load draws, the amount of time it runs each day, and the number of days it’s used each week.
Certain loads may only run a few times a week, whereas others may run daily. By averaging out the loads over a week’s time, you can establish a consistent pattern of energy consumption. Use the following equation:
Energy (in watt-hours) = (Watts x Hours per day x Days per week) ÷ 7 days per week
When estimating weekly energy consumption, include all the watts drawn. For example, if you’re looking at lighting, don’t just calculate the energy based off one light — look at all the lights that will be on at the same time.
Here’s another example: Pretend that the washing machine isn’t run each day in your client’s home. If you calculated the washing machine’s energy consumption based on the days it runs, you’d end up with a value that’s higher than normal. By instead averaging out the washing machine’s energy consumption over the course of a week, you wind up with a daily energy consumption that’s slightly higher than the reality for the days the washer doesn’t run and slightly lower than the reality for the days it does run. This results in a good approximation for the week.
Imagine a washing machine that draws 175 W for 45 minutes. If it’s run four days a week, you can determine the average daily energy value by multiplying the power draw by the number of hours to get 175 W x 0.75 hrs = 131 Wh, or 0.131 kWh
So the washing machine uses 0.131 kWh each time it’s run, and it’s on four days per week. Therefore, the average daily energy consumption is 131 Wh x 4 days ÷ 7 days = 75 Wh per day.
With all the loads that clients want their battery-based systems to support, tracking the individual energy-consumption amounts can get confusing. I recommend using a spreadsheet to help keep all the calculations straight.
Having a spreadsheet also makes it easier to make major changes should the client decide any are necessary. Keep your load-analysis spreadsheet simple, like the one in Figure 1.
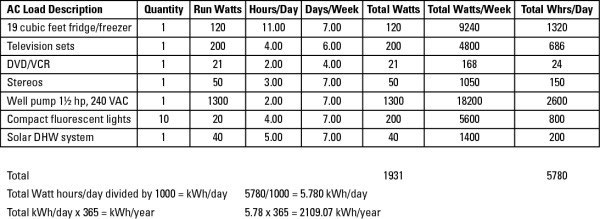
In this chart, you see a few loads that are to be powered by a battery-based PV system. The first column denotes the itemized loads; the second column indicates how many of each item there are. The third column indicates the power draw (in watts) of an individual load; the next two columns represent the number of hours per day and the number of days per week each load is estimated to run, respectively.
The second-to-last column denotes the number of watts each load draws multiplied by the number of items; the final column calculates the average daily energy consumption of each load by multiplying the total run watts by the number of hours per day multiplied by the days per week and then divided by seven.
To get a good feel for the table, look at the television row. This client has a nice new big-screen TV that draws 200 W when it’s on, and she has admitted that the TV is on for 4 hours on the days that her family watches it. So each day anyone in the client’s family watches TV, the set consumes 200 W x 4 hours = 800 Wh.
But there’s one day every week that the family doesn’t watch TV, so to determine the average daily energy consumption for the week, you multiply the 800 Wh by the 6 days per week the client’s family actually watches it and divide that number by 7 days per week. Doing so puts the average daily energy consumption for the TV at 686 Wh, or 0.686kWh.
If your client wants to use any DC appliances, such as lighting or refrigeration, you need to account for the energy consumption for those loads as well. You calculate this consumption the same way you do for AC loads, but keep the numbers separate in your tables; you have to account for the efficiency losses associated with converting the DC in the batteries to AC for the AC loads.
After you take the efficiency losses into account, you can add the two values together to find the total energy consumption of all the loads. The inverter itself is a load that’s always present unless advanced energy-management techniques are used when programming the inverter.
This load isn’t large, but if you don’t consider it as being left on 24 hours a day, the inverter’s idle draw can have a significant effect on the rest of your system sizing and the overall performance of the system. You can find the exact amount of energy an inverter draws when it’s sitting idle by looking at the manufacturer-provided specification (spec) sheet. Simply grab the spec sheets of the inverters you’re most likely to use, and estimate each inverter’s power consumption from its respective spec sheet.