Magnetostrictive Sensor Working Principle
The magnetostrictive effect relates a material’s elastic state to its magnetic state. Nearly all FM materials such as iron, nickel, cobalt, and their alloy, exhibit a change in size and shape resulting from magnetization change. This effect is known as Joule effect.
Joule effect can be understood by considering an FM material consisting of many tiny, oval shaped, permanent magnets. When the material is not magnetized, the domains are randomly arranged. If the material is magnetized, the domains are oriented with their axes approximately parallel to one another.
As a result, the overall dimension of the material changes (expands or contracts, depending on the direction of the magnetic field applied) as shown in Figure 1.
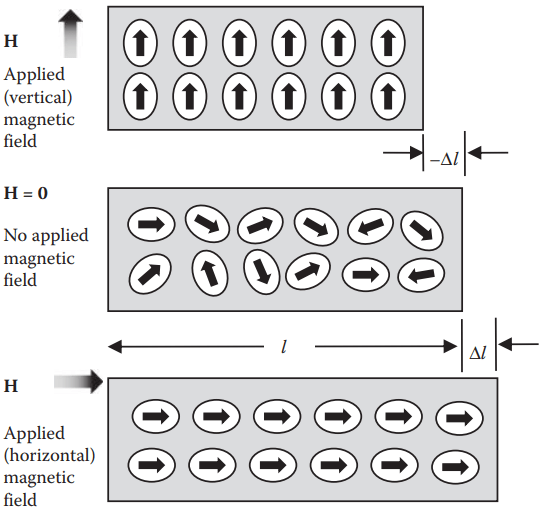
Inversely, applying a force to an FM material will cause a change in its magnetization. This effect is called Villari effect.
Additional magnetostrictive effects are Wiedemann and Matteuci effects. When a current carrying FM rod is placed in a longitudinal magnetic field, the rod experiences twisting. This phenomenon is called Wiedemann effect. Inversely, twisting a magnetostrictive element or a magnetized wire causes a change in magnetization (Matteuci effect).
Magnetostrictive Sensor Working Principle
A magnetostrictive sensor operates based on Joule, Villari, Wiedemann, or Matteuci effect. On the one hand, the stress created by an applied force or torque alters the permeability of a magnetostrictive material, causing its magnetization change; on the other, an applied magnetic field changes the magnetic state of a magnetostrictive material, resulting in a variation in its elastic constant.
Depending on whether an excitation is used in the measurement, magnetostrictive sensors can be classified as active, passive, or hybrid sensors. Active sensors use an internal excitation of the magnetostrictive element to facilitate the measurement. Passive sensors “passively” rely on the change in the magnetostrictive material properties to perform the measurement. Hybrid sensors use a magnetostrictive element to actively excite or change another magnetostrictive element to make the measurement.
Applications of Magnetostrictive Sensors
The latest magnetostrictive sensor development focuses on using giant magnetostrictive material (such as Terfenol-D), magnetostrictive amorphous wire and thin films. These developments have resulted in successful sensor designs, including hearing aids, load cells, accelerometers, motion and proximity sensors, torque sensors, stress or force sensors, vibration sensors, magnetometers, flow meters, and many more. The following sections present several design and application examples of magnetostrictive sensors.
Magnetostrictive Position Sensor: A magnetostrictive position sensor usually consists of a magnetostrictive wire (or called waveguide, serving as the measuring element), a permanent magnet and a pickup coil (converting the mechanical pulse into an electric signal). The pickup coil can be a single coil, a dual coil, an FM recording tape, or a piezoelectric element.
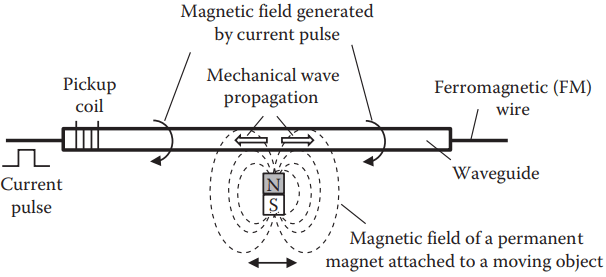
Figure 2 shows a magnetostrictive position sensor. A current pulse is applied to the FM wire where it generates a circular magnetic field around the wire. The permanent magnet (rectangular or ring shape) is connected to a moving object whose position is to be measured.
This permanent magnet’s magnetic field interacts with the magnetic field of the current pulse, resulting in an elastic deformation of the waveguide in a form of mechanical pulse (Joule effect). This wave travels along the waveguide in both directions with sound speed.
At one end of the guide, a pickup coil detects this wave and converts the strain change into either an electrical signal or a magnetic field change (Villary effect). At the other end of the guide, the unused pulse is attenuated by a damping module to prevent further interference with returned/reflected pulse.
The position of the object thus can be accurately determined by using the time elapsed, t, from emitting the current pulse to receiving the strain pulse as follows:
Position of the Object = vst/2
Where vS is the speed of sound (vS = 340 m ⋅ s−1 ).
The sensors that are based on the time elapsed are often called magnetostrictive delay lines (MDL). To ensure the accuracy of the output, the actual speed of sound in the waveguide is usually determined or calibrated using a laser interferometer for each sensor at the final stage of production.
To extend the measurement range or to track multiple target positions, a multiposition magnetostrictive sensor is developed. It can monitor multiple targets in a one-time setup and can measure a stroke range greater than 5 m with a resolution down to 1 mm.
Magnetostrictive Level Transmitter: Figure 3 shows a KSR’s magnetostrictive level transmitter. It consists of a magnetostrictive wire (held under tension inside a guide tube), a float (fitted with permanent magnets) that can move along the guide tube as the liquid level changes, and the sensor electronics.
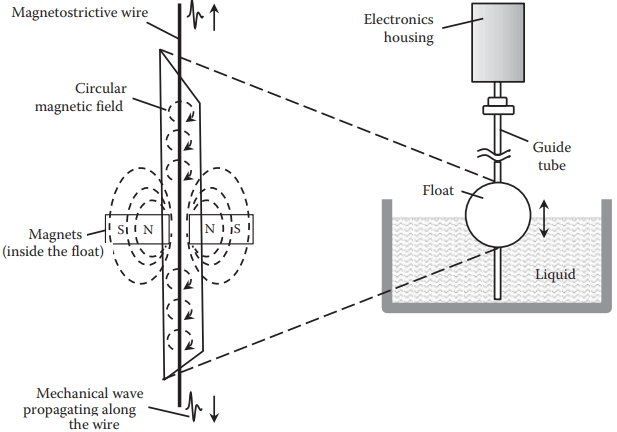
A current pulse is released to the wire and generates a circular magnetic field along the length of the magnetostrictive wire. When the pulse reaches the float, the pulse’ circular magnetic field interacts with the float’s magnetic field, generating a torsional stress pulse in the wire.
A piezoceramic converter at the end of the wire converts this stress into an electrical signal. By measuring the elapsed transit time from the starting point of the current pulse to the receiving point of the resultant stress wave, the float position can be determined with high accuracy. This system works only if the auxiliary column and chamber walls are made of nonmagnetic material.
Magnetostrictive Force/Stress Sensors: Many magnetostrictive force and torque sensors have been developed based on Villary effect, whereby the force or torque being measured acts on the MDL and interrupts the emitted acoustic signal. The change in the acoustic wave captured by a receiver coil is thus related to the force or torque applied. The delay line can also be embedded in a fiber glass channel. The channel bends under an applied force, which changes the optical path or index that can be measured (optical-magnetostrictive force sensor).
Figure 4a shows an example of a magnetostrictive force sensor. The sensor consists of two coils (each surrounds a magnetostrictive core): one for excitation by passing a current to generate an oscillating magnetic field; the other for detection. Both cores are held in place by rigid end pieces. An applied force will cause a strain change in the magnetostrictive cores, resulting in a change in the core’s magnetization that can be converted into a change in the output voltage by the detection coil.
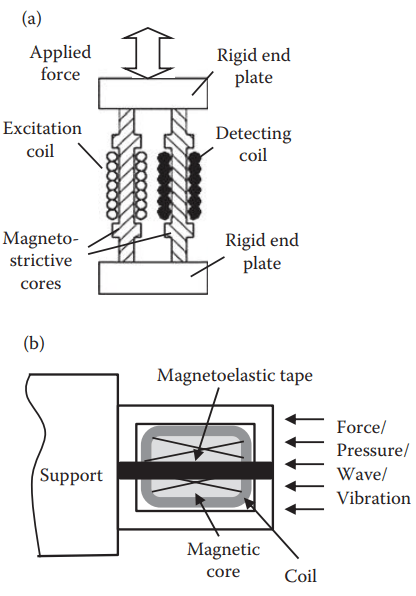
In Figure 4b, the sensing element is a magnetoelastic tape. When a force, pressure, wave, or vibration presents, the permeability of the tape changes, causing the bias magnet flux lines change. The output voltage generated in the detecting coil is proportional to the applied parameter.
This method can detect strains less than 10−10 and it is suitable for vibration or shock measurement, as well as for very weak seismic activity monitoring. Compared with conventional force transducers, such as strain gages, magnetostrictive force sensors are simpler, more rugged, and relatively inexpensive.
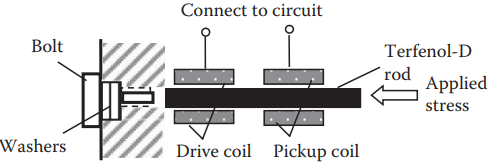
Figure 5 is a Terfenol-D stress sensor. It has a 2″ long and 1/4″ diameter laminated Terfenol-D rod (Tb0.3Dy0.7Fe1.9) placed inside two coils. One coil is a multilayer 1100 turn drive coil, the other is a single-layer 110 turn pickup coil. A magnetic field is produced by passing a current to the drive coil. Additional magnetic field is provided by a slit, cylindrical permanent magnet surrounding the coils.
A mechanical prestress is provided by inserting washers in series, and this stress can be adjusted by turning a threaded bolt to meet the need of the measurement. The stress to be measured is applied on the right end of the rod that will change the magnetic flux density B.
The pickup coil will detect this change and convert it into a measurable voltage that is proportional to the time derivative of B. Notice that this Terfenol-D sensor measures the time derivative of the load rather than the load itself.
Magnetostrictive Torque Sensors: Many torque sensors are designed based on magnetoelastic phenomenon because it offers noncontact measurement, high sensitivity, small size, and durability.
A typical magnetostrictive torque sensor includes an FM shaft supported in a housing, a detecting coil, and a thin layer of magnetostrictive material coated on the peripheral surface of the shaft. The detecting coil is usually fixed to the inner wall of the housing (stator), and a predetermined air gap exists between the stator and the shaft, as shown in Figure 6.
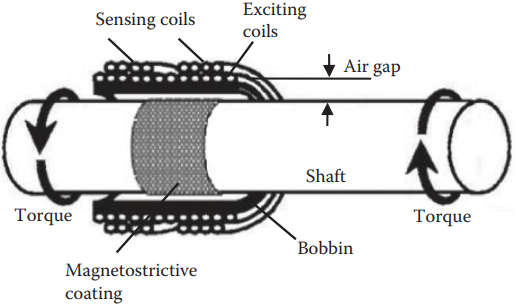
Several designs of magnetostrictive torque sensors are shown in Figure 7. In Figure 7a, the drive coil and the detection coil are wound around a C-shaped FM core placed near the shaft (without contact) and oriented to 45° from the shaft axis.
The current passing through the drive coil generates a magnetic field that penetrates the shaft. If a torque is applied to the shaft, stress will be developed in the shaft at 45° from the shaft axis, resulting in a change in permeability of the shaft, hence a change in the magnetic field. The changing magnetic field induces a current in the pickup coil that is proportional to the applied torque. Two factors can affect the sensor output: the air gap between the shaft and the sensor core and current in the drive coil.
To eliminate the influence of the variation in the air gap due to eccentric shaft motion, three sensors are placed equally around the circumference of the shaft Figure 7b. In this design, the drive coil (with a laminated silicon steel core to prevent eddy currents) and the pickup coil (with a laminated permalloy core) are mounted at 90° angle to each other in a ceramic holder—one coil is parallel and the other coil is perpendicular to the axis of the shaft.
The shaft is magnetized in circumference direction under the magnetic field generated by the exciting coil. If no torque is applied, the direction of the magnetization vector coincides with that of the shaft circumference.
When a torque is applied, stress is generated in the shaft, which reorients the magnetization vector toward the direction of the stress. This magnetization vector change is detected by the pickup coil through electromagnetic induction.
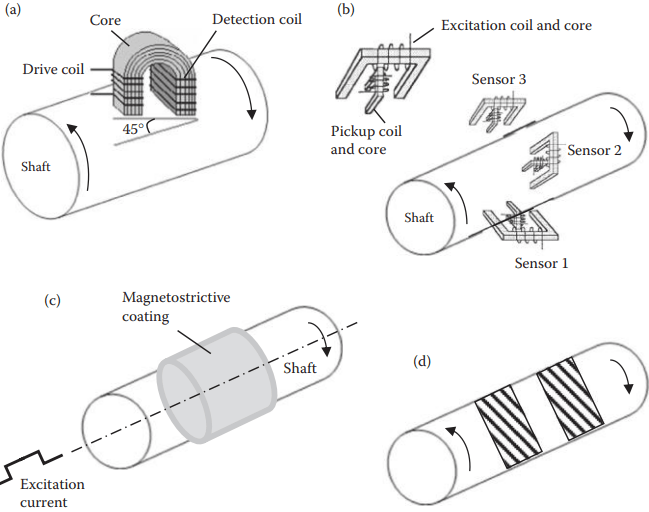
The torque sensor shown in Figure 7c has a magnetostrictive material (e.g., Ni, FeNi, or FeCoNi) rigidly coated to the shaft. The coating is magnetized by passing a pulsed current through the shaft. When a torque is applied to the shaft, the magnetization in the coating reorients and becomes increasingly helical as the torque value increases. This reorientation is detected by the sensing coil.
Some shaft materials require knurl grooves in oblique direction to the shaft axis (see Figure 7d), so that a magnetostrictive layer can be rigidly deposited (by plating or thermal spraying) in the outer periphery of the shaft and adhere to the shaft’s surface to enhance the output signals.
Magnetic Field Sensor: If a magnetoelastic thin film or coating is placed on a SAW (surface acoustic wave) device, any magnetic fields nearby will cause stress to occur across the boundary layer due to the magnetostrictive effect. This stress will alter the velocity of the SAW. Hanna has reported a resonator-type SAW magnetic sensor based on SAW propagation in magnetoelastic films. The relationship between resonant frequency and magnetic field of the sensor was nonlinear but a high sensitivity was achieved.
Xiao et al. developed a similar sensor. They used a giant magnetostrictive material Terfenol-D and a SAW resonator. The resonant frequency of the SAW resonator varies with the stress induced by an applied magnetic field. Thus, the strength of the magnetic field can be determined through the variation of the resonant frequency. The static sensitivity of the sensor can reach 341.6 Hz ⋅ Oe−1 ; the resolution of the magnetic field can reach 10−6 T; the resolution of frequency detection is 1 Hz, and the frequency stability of the SAW resonator is 0.1 × 10−6.
Magnetostrictive Fiber-Optic Magnetometers: Numerous magnetostrictive and fiber-optic combined magnetic field sensors have been developed. Wang et al. used an FM polymer (WCS-NG1) and the magnetostrictive film coated on an optic fiber for magnetic field detection. An applied magnetic field causes the magnetostrictive film to deform and strain the optic fiber.
As a result, the length of the optical path of the laser changes. An interferometer is used to measure the phase changes. A magnetostrictive fiber-optic sensor has a number of advantages:
- The optical technique provides high sensitivity to its measurand and is relatively simple to fabricate magnetostrictive coating on the optical fiber. Therefore, magnetostrictive effect is often used for the fiber optics magnetometer.
- The optical technique also prevents RF (radio frequency) interference that is common in typical electromagnetic-type sensors. Some magnetostrictive fiber-optic sensors are reported of having the resolution of 3 × 10−11 Oe at DC to low frequency (<1 Hz).
- Compared to other conventional magnetostrictive sensors, a magnetostrictive fiber-optic sensor is much less complex and relatively smaller in size.
The magnetostrictive fiber-optic method is specially useful in systems that already have optical fibers, for example, measuring the magnetic field along optical-fiber cables. Some sensitive magnetostrictive sensors also use a piezoelectric element to detect the length variation. A schematic diagram of using a magnetostrictive fiberoptic sensor to measure small-amplitude RF magnetic field distributions is shown in Figure 8.

Thanks for reading about “magnetostrictive sensor working principle”.